Microplastics Case Studies
This section presents several case studies illustrating various aspects related to MP. There are six case studies:
A.1 California Approach for Microplastics—describes the legislation and other activities the State of California is taking related to MP.
A.2 Consequences of Microplastics on Various Ecological Endpoints in the Chesapeake Bay and Its Tributary Estuary, the Potomac River—discusses the effects of MP on three organisms found in the Chesapeake Bay: the hooked mussel, blue crab and the striped bass.
A.3 Impact of Disposable PPE and Single-Use Plastic Items During the COVID-19 Pandemic —explores the effects of the increase in single-use plastics related to the COVID-19 pandemic.
A.4 Nurdles along the Gulf Coast—describes a notable spill of plastic nurdles into the Mississippi River.
A.5 Effects of 6PPD-quinone on Coho and Chum Salmon—evaluates TWP impact on acute mortality in coho and chum salmon and details mitigation efforts to decrease the transport of tire particles to surface waters.
A.6 Washing Machine Filters Reduce Microfiber Emissions to Aquatic Ecosystems—policy brief prepared for Toronto, Canada.
References are provided for each case study.
A.1. California Approach for Microplastics
Microplastics Legislation:
The State of California has been very active in passing legislation aimed at addressing MP in the environment. Adopted bills have regulated the discharge of MP to the environment, instituted bans on MP-containing personal care products, required the establishment of a regulatory definition of MP in drinking water, required the standardization of methods for sampling and analysis, and led to the establishment of a statewide strategy for dealing with MP. All of this work has been done through collaboration among state boards, departments, nongovernment agencies, environmental advocacy groups, research institutions, and other scientific organizations to evaluate and determine the best scientifically defensible approaches to tackling this emerging and persistent environmental concern.
In 2007 and 2015 the first two such pieces of legislation were passed. In 2007, Assembly Bill 258 (AB 258) was passed. This was California’s first piece of legislation that explicitly addressed MP. The bill required the implementation of a program to regulate discharges of preproduction plastics, defined as plastic resin pellets and powdered coloring for plastics, from point and nonpoint sources (State of California 2007). AB 888 was passed in 2015; it prohibits any person from selling or offering for promotional purposes in California rinse-off personal care products containing plastic microbeads that are used to exfoliate or cleanse (State of California 2015). AB 888 also defined plastic microbeads as an intentionally added solid plastic particle measuring five millimeters or less in every dimension.
Two major pieces of legislation were passed in 2018 that are responsible for the major efforts currently underway in California to address MP in the environment. Senate Bill 1263 (SB 1263) requires the Ocean Protection Council (OPC) “to adopt and implement a Statewide Microplastics Strategy, related to MP materials that pose an emerging concern for ocean health” (State of California 2018a). SB 1422 required the State Water Resources Control Board (SWRCB) to adopt a definition of MP in drinking water (Figure A.1-1), adopt a standard methodology to be used in the testing of drinking water for MP, and established requirements for four years of testing and reporting of MP in drinking water, including public disclosure of the results (State of California 2018b).
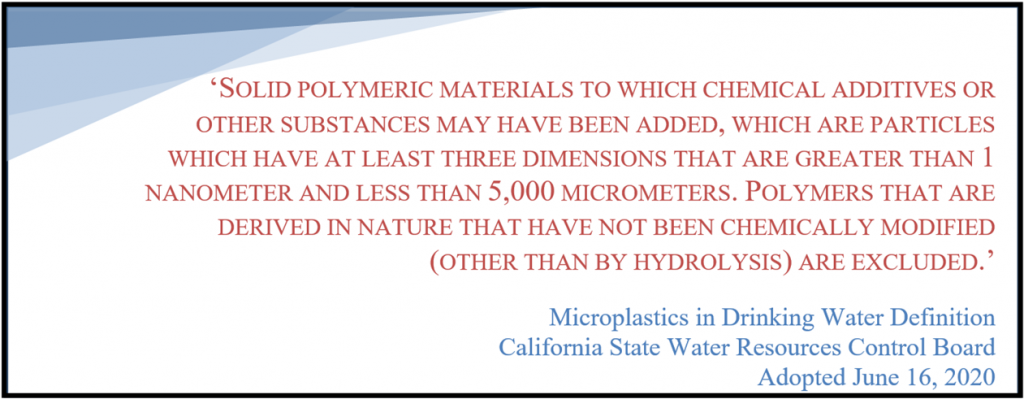
Source: CA SWRCB (2020)
California’s Statewide Microplastics Strategy:
OPC released California’s Statewide Microplastics Strategy in February 2022 2022 (California Ocean Protection Council 2022). The strategy uses a two-track approach with one focusing on current solutions that can be implemented today to reduce MP in the environment and the second one focusing on the need for science to inform future action (Figure A.1- 2). Numerous workshops and studies were conducted aimed at strengthening the scientific foundation on which to build the Statewide Microplastics Strategy.
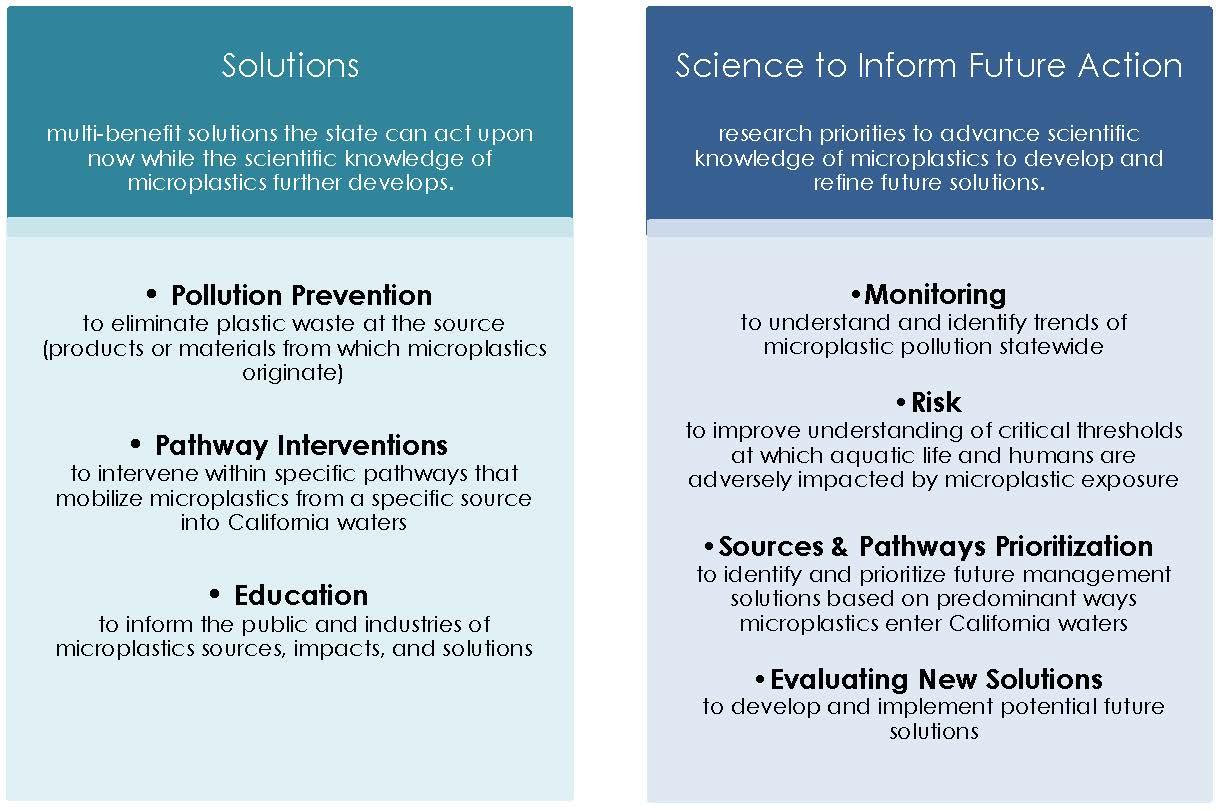
Figure A.1- 2. Two-track approach from the Statewide Microplastics Strategy.
Source: California Ocean Protection Council (2022)
Through funding by OPC, the Ocean Science Trust organized a scientific advisory team and an MP Working Group to evaluate the risks that MP pose to marine ecosystems (Brander et al. 2021). The working group recommended a precautionary framework (see Figure A.1-3) for evaluating risks associated with MP. The precautionary framework was recommended to empower the state to take immediate action, even under existing scientific uncertainties related to toxicity. Some of the recommendations include taking a particle approach rather than a toxicological approach in assessing risk with a focus on particle morphology (microfibers and fragments) and highlighting specific polymer types such as microfibers and tire and road wear particles. The working group identified stormwater runoff, aerial deposition, and wastewater as the primary MP fate and transport pathways. It also placed a heavy emphasis on the importance of source reduction in reducing and preventing MP pollution.
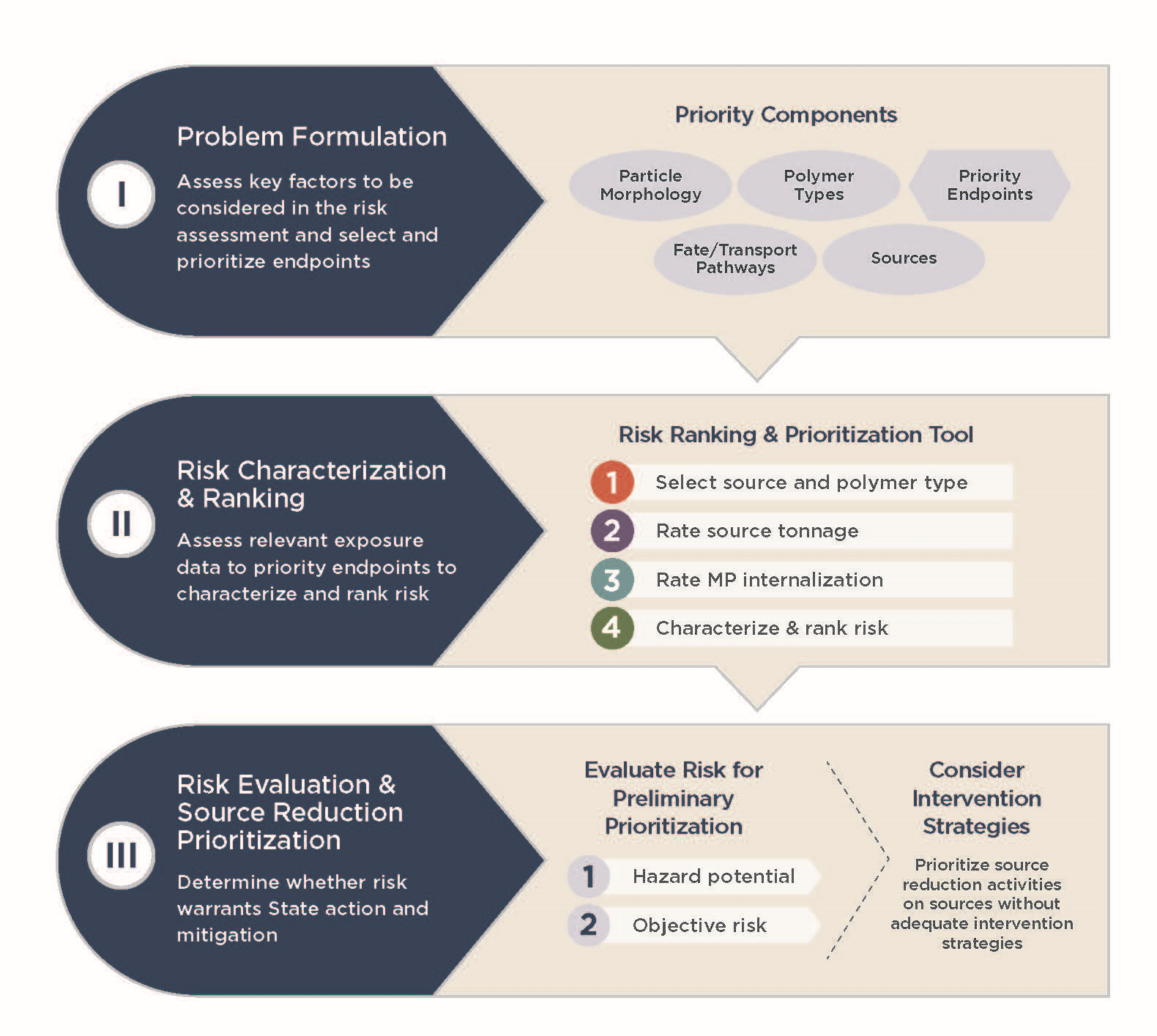
Figure A.1- 3. Precautionary risk assessment framework for MP with phases on the left and steps and recommendations on the right.
Source: Brander et al. (2021)
Measurement Methods Workshop:
The Southern California Coastal Water Research Project (SCCWRP) and the San Francisco Estuary Institute (SFEI) have played a critical role in California’s efforts regarding MP. SCCWRP has hosted multiple workshops to bring together international experts to help address the mandates of SB 1263 and SB 1422. In spring 2019, SCCWRP hosted the Microplastics Measurement Methods Workshop, which was the first step by the SWRCB toward the development of standardized methods for evaluating MP in drinking water. Twenty-two laboratories in six different countries participated in an interlaboratory study to assess methods for accuracy, precision, and repeatability (De Frond et al. 2022). In September 2021, two standardized methods were released for the extraction and measurement of MP in drinking water: infrared spectroscopy (CA SWRCB 2021a) and Raman spectroscopy (CA SWRCB 2021b). Laboratory accreditation was made available for these two methods in 2022, fulfilling two of the requirements of SB 1422.
Health Effects Workshop:
A Microplastics Health Effects Workshop was hosted by SCCWRP and SFEI along with the University of Toronto through a series of webinars in fall 2020 (SCCWRP 2021). This workshop, developed in coordination with the SWRCB and OPC, brought together international experts to discuss thresholds at which biological effects are likely triggered in both humans and aquatic organisms at ambient concentrations. The outcome of this workshop is a series of papers published as a collection in Microplastics and Nanoplastics (SCCWRP 2022b).
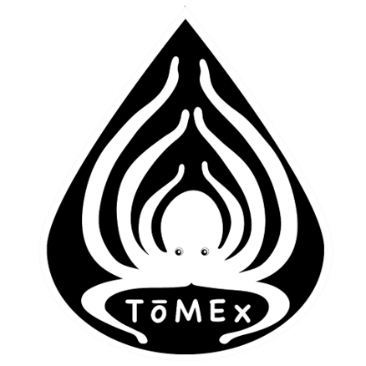
Figure A.1- 4. Toxicity of Microplastics Explorer (ToMEx) logo.
Source: (SCCWRP 2022a)
One of the biggest hurdles in the development of these thresholds was evaluating the large number of publications from just the last decade that evaluate risk to both humans and ecological receptors from exposure to MP. The Toxicity of Microplastics Explorer (ToMEx) was developed to aid in the development of risk thresholds. ToMEx consists of an MP toxicity database and allows visualization and analysis of data through the R Shiny application (SCCWRP 2022a). There are separate databases for aquatic organisms and human health. There are more than 70 unique variables extracted or derived from each study in the database, which currently includes 162 publications on aquatic organisms and 55 publications on human health (Thornton Hampton et al. 2022). A “Data Submission Portal” allows researchers to submit their data from peer-reviewed publications for inclusion in the ToMEx application, so the database stays current with the state of the science (Figure A.1-4).
Another outcome from the Health Effects Workshop was the development of a nonregulatory screening level for monitoring MP in drinking water. A tiered approach was used to screen the available in vitro and in vivo mammalian MP studies into those that were fit for the purpose of developing a drinking water standard (Coffin, Bouwmeester, et al. 2022). The expert group ultimately decided to include only in vivo studies involving oral exposure to MP. Of the twelve available studies, 83% were focused on exposure to a single polymer type (PS spheres). Ultimately, there was too much uncertainty in the data to establish a health-based screening level for regulatory purposes. They did, however, establish a nonregulatory screening level of 0.3 micrograms per liter (µg/L) or 5.7 x 105 particles/L specifically for 0.5 µm PS spheres.This screening level was used to estimate that 1,000 liters of drinking water was the appropriate sampling volume for future monitoring activities.
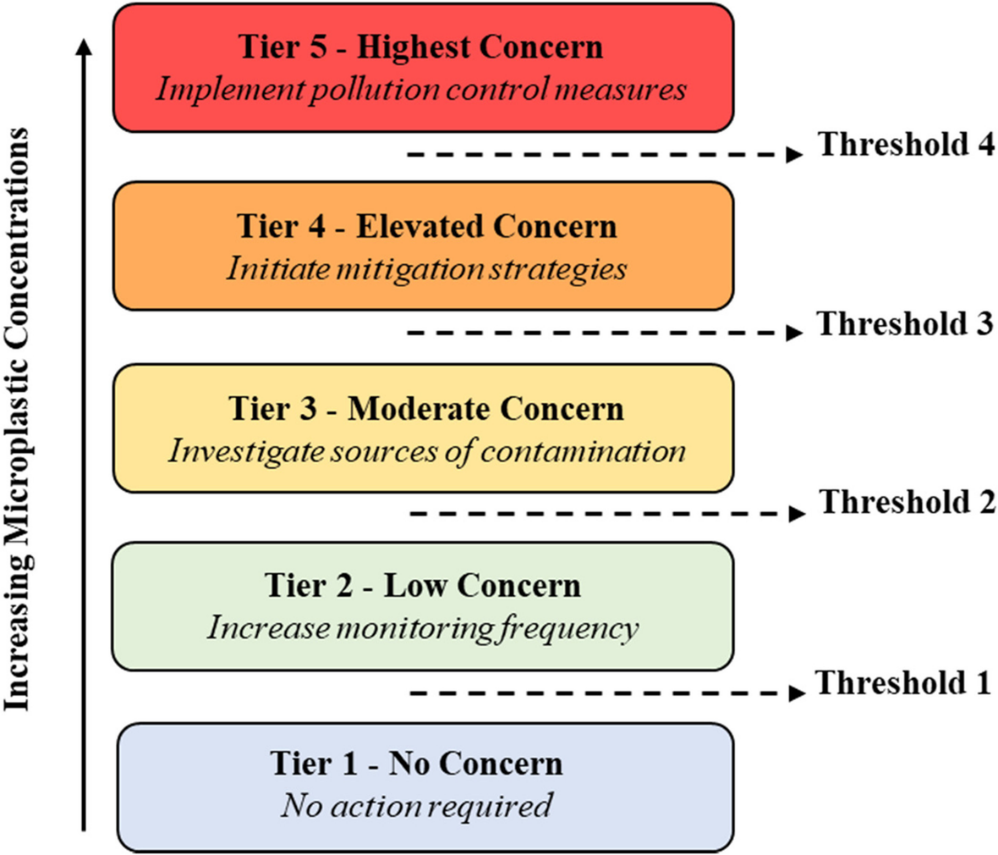
Figure A.1- 5. Aquatic ecosystem health-based thresholds for microplastics.
Source: (Mehinto et al. 2022)
The SFEI and 5 Gyres Institute conducted a comprehensive study of MP pollution in San Francisco Bay (Sutton et al. 2019). To date, this is the most comprehensive MP data set in the state and it helped inform the Statewide Microplastics Strategy (California Ocean Protection Council 2022). This was the first of its kind for a major estuary and established many best management practices for sampling (Miller et al. 2021). Stormwater was found to be the dominant pathway for MP transport from urban areas to receiving waters. Microplastics in stormwater were mostly tire wear particles and fibers. Microplastic concentrations in wastewater were significantly lower compared to stormwater runoff. SFEI further developed conceptual site models to aid in the development of management strategies specific to key MP sources to urban runoff through a study funded by the OPC (Moran et al. 2021). SFEI focused on the main sources identified in the urban runoff from the San Francisco Bay study: tire particles, fibers from textiles, single-use plastic foodware, and cellulose acetate fibers from cigarette butts. The MP monitoring data from the San Francisco Bay study (Zhu, Munno, et al. 2021) were compared to four health-based thresholds (Figure A.1-5) based on food dilution established for aquatic ecosystems (Mehinto et al. 2022) to characterize the exposure risk in San Francisco Bay, California (Coffin, Weisberg, et al. 2022), which is illustrated in Figure A.1-6.
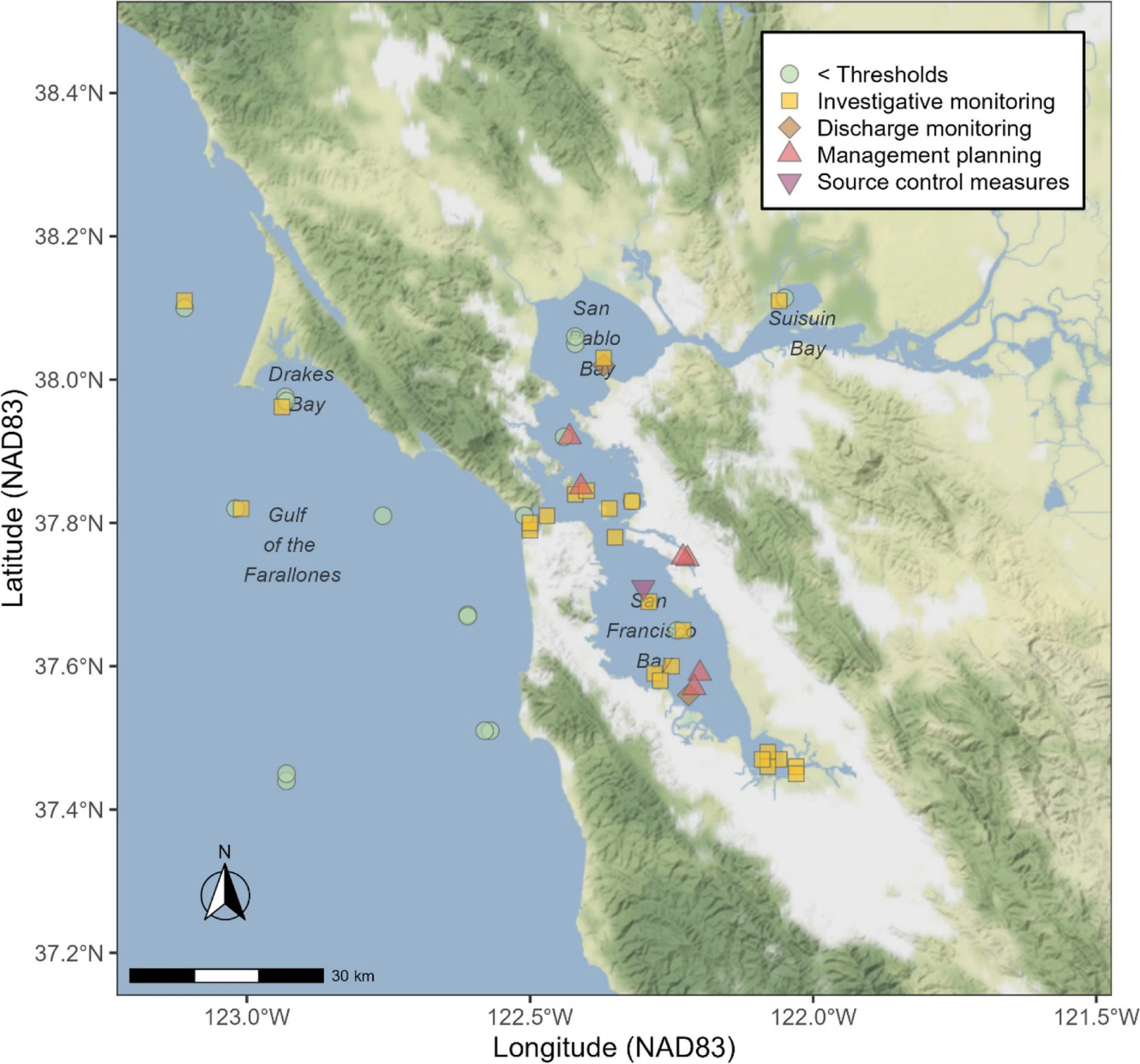
Figure A.1- 6. Health-based aquatic risk threshold exceedances in San Francisco Bay.
Source: Coffin, Weisberg, et al. (2022)
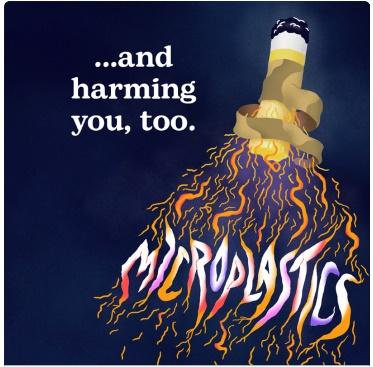
Figure A.1- 7. Illustration from The Little Big Lie media campaign.
Source: California Department of Public Health (2022b)
The Little Big Lie:
Cigarette butts are one of the most commonly littered items (Bergmann, Gutow, and Klages 2015). In 2022 the California Department of Public Health (CDPH) launched a media campaign “The Little Big Lie,” which focuses on MP pollution caused by cigarette filters. The campaign includes a website (California Department of Public Health 2022b), radio advertisements, and a video (California Department of Public Health 2022a) (Figure A.1-7). Additionally, CDPH contracted the development of a white paper on tobacco product waste in California (Novotny et al. 2022), which further discusses the contribution of electronic smoking devices to plastics pollution. The white paper helped inform the Statewide Microplastics Strategy, which includes a recommendation to “prohibit the sale and distribution of single-use tobacco products…including cigarette filters, electronic cigarettes, plastic cigar tips, and unrecyclable tobacco product packaging” (California Ocean Protection Council 2022).
California Safer Consumer Products Program:
The California Department of Toxic Substances Control (DTSC) Safer Consumer Products (SCP) program has also signaled an interest in MP in their Three-Year Priority Product Work Plan (2021–2023) (CA DTSC 2020). SCP identifies priority products, which are chemical-product combinations that pose a significant or widespread adverse effect. The work plan states that special consideration will be given to products that “release microplastics to the environment during the use or end-of-life stages of the product’s life cycle.” MP were also the focus of the November 2021 Green Ribbon Science Panel (GRSP) meeting (CA DTSC 2021). The GRSP is a panel of experts that advises DTSC on matters related to green chemistry. SCP posed the question to the GRSP if microplastics should be added to the Candidate Chemical List. A product must include a chemical on the Candidate Chemical List to be named as a priority product.
Conclusion:
While California has been very involved in addressing the issue of MP in the environment, there is still much more work to be done. The SWRCB is currently overseeing the required four years of monitoring of MP in drinking water and will continue to evaluate new toxicological studies so that health-based screening levels may be developed. The Statewide Microplastics Strategy includes numerous recommendations to reduce MP in the environment through pollution prevention and pathway interventions. Education and outreach to the public will play a critical role in source reduction for this global issue.
A.2. Consequences of Microplastics on Various Ecological Endpoints in the Chesapeake Bay and Its Tributary Estuary, the Potomac River
Introduction
The increasing prevalence of MP found in essentially every habitat on earth, from oceans and estuaries to ice and sediment cores, raises great concern regarding the ingestion of MP by animals and bioaccumulation within food webs (Bikker et al. 2020, Woodall et al. 2014). Data collection from a study done by Yonkos et al. (2014) found that concentrations of MP had a positive correlation to nearby population and land use factors, with their accumulation at its densest downstream of major cities. This is especially true for rivers like the Potomac River, which act as major vectors of transport of MP to oceans and other larger water bodies (Du et al. 2020). In the case of many urban areas, including Washington, D.C., the bulk of MP were found downstream, with stormwater and precipitation picking up a significant amount of debris and distributing it to other water sources. As one of North America’s largest estuaries, Chesapeake Bay is a coastal plain with a long history of water quality degradation (Figure A.2-1). Excess chlorophyll and nutrients have led to dwindling flora and fauna and a decline in water clarity, and the bay has the potential to become a large sink for MP (López et al. 2021). As stormwater flows through the watershed and into the bay, it picks up litter and promotes plastic erosion, resulting in MP pollution (Cole et al. 2011). The Potomac River, one of the two largest tributaries to the Chesapeake Bay, contributes about 11% of the bay’s MP particles.
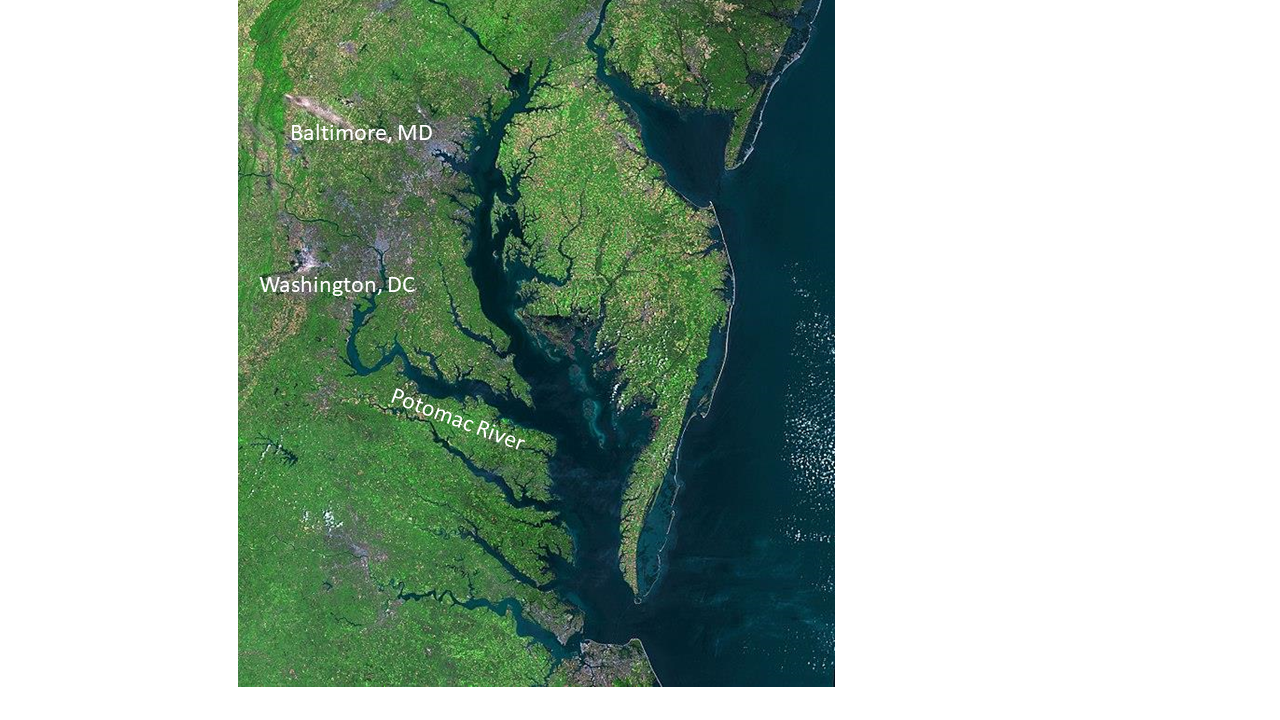
Figure A.2- 1. Aerial view of the Chesapeake Bay, showing Washington, D.C., Baltimore, MD, and the Potomac River.
Source: NASA/USGS Landsat 5
With the continued increase in plastic waste entering the environment, various ecological endpoints have been negatively impacted. Studies conducted on several key species demonstrate the consequences of these interactions with MP, such as ingestion and entrapment. Due to the estimated 4.8–12.7 million tons of MP released into the marine environment worldwide every year, there is mounting concern over the buildup of MP and their ability to infiltrate every chain in the food web, ultimately ending up on the plates of consumers (Jung et al. 2018, Moser et al. 2020).
Adverse Effects of Microplastics on Ecological Endpoints
Hooked Mussel
Over the past several decades, the Chesapeake Bay’s mussel and oyster populations have been reduced to about 1% of their original total due to overfishing and pollution (Lipcius and Burke 2018). The repercussions of MP pollution have become increasingly apparent as studies have shown declines in marine bivalves’ filtration efficiency from MP ingestion. The hooked mussel (Ischadium recurvum) plays a significant role in cleaning the Chesapeake Bay and maintaining its water quality (Betsill, Gonzalez, and Woods 2019). The mussels’ filtration system filters toxins out of the water, resulting in clearer, less contaminated water. Their modified gills, known as ctenidia, are able to absorb dissolved oxygen for cellular respiration and to sift food, such as phytoplankton, plant matter, and bacteria. Additionally, their gills are lined with thread-like organelles called cilia, which further aid in the sorting and filtering (Betsill, Gonzalez, and Woods 2019). Unfortunately, hooked mussels are unable to break down plastics and other polymers no matter the size because they lack the enzymatic pathways necessary to complete the process. Due to this, mussels ingest MP but cannot eliminate them, resulting in plastic getting incorporated into the bivalve’s body, from their gills to their digestive gland. The accumulation of plastics within the hooked mussel leads to loss of energy, reduction of efficiency, and hindrance of filtering abilities (Betsill, Gonzalez, and Woods 2019).
The deterioration of water quality has been noticeable in the Chesapeake Bay and its tributaries, including the Potomac River, and has been accelerated by human pollution and waste. Increased sediment and nutrient loads have been transported and deposited into the Chesapeake Bay. Due to their buoyancy, extreme durability, and synthetic polymers, MP are able to easily accumulate in beaches and other coastal areas, float, and combine with sediments (Du et al. 2020). Furthermore, once MP in the sediment aggregate together in an open water environment, they can quickly escape the water column, transporting to shores and organism habitats (Vermaire et al. 2017). Although the bay has been historically filled with oysters and mussels to combat water quality degradation, the decline in marine bivalve populations and increase in waste have brought about a continued decline in water and biological resource quality, with the Potomac River estuary mirroring this condition with high nitrogen loads and eutrophication (Bricker, Rice, and Bricker 2014).
Blue Crab
Serving as both predator and prey, the blue crab (Callinectes sapidus) is a vital keystone species in Chesapeake Bay. It maintains the food web’s ecological balance while also acting as a decomposer to clean up the sea bottom (Figure A.2-2). However, according to the annual Blue Crab Winter Dredge Survey, the number of blue crabs in Chesapeake Bay has hit a record low of 227 million, estimated, in 2022. This is a drastic decrease from previous years, with blue crab populations typically averaging 300–700 million (MD DNR 2022)). Much of this decline can be attributed to overfishing, habitat destruction, and plastic pollution. Unpublished studies by Cohen and Boettcher (University of Delaware) suggest that movements of blue crabs through their life cycle greatly overlap with MP transport. Adult crabs release their larvae at the mouth of the bay and larvae are pushed out into the open ocean where they have time to develop and eventually make their way back into the bay to rejoin the adult population (Cohen 2020). However, the larvae’s path mirrors that of MP, where the physical forces of wind and water that are pushing the larvae out are the same forces transporting MP in the water. Given how early the blue crab larvae are exposed to this plastic pollution, their energy consumption, growth, and survival are all negatively impacted (Cohen 2020). Especially when considering the 35–40 days it takes for blue crab larvae to develop from eggs into first-stage crabs, they are particularly susceptible to higher MP intake and concentrations, potentially lowering their overall oxygen consumption rates and impairing their growth to adulthood (Cohen 2020). Moreover, the loss of marshes and abundant communities of seagrasses and from plastic pollution, especially in the shoal areas of the Chesapeake and Potomac, have been reduced the nursery and feeding habitat for blue crabs (Kemp et al. 2005).
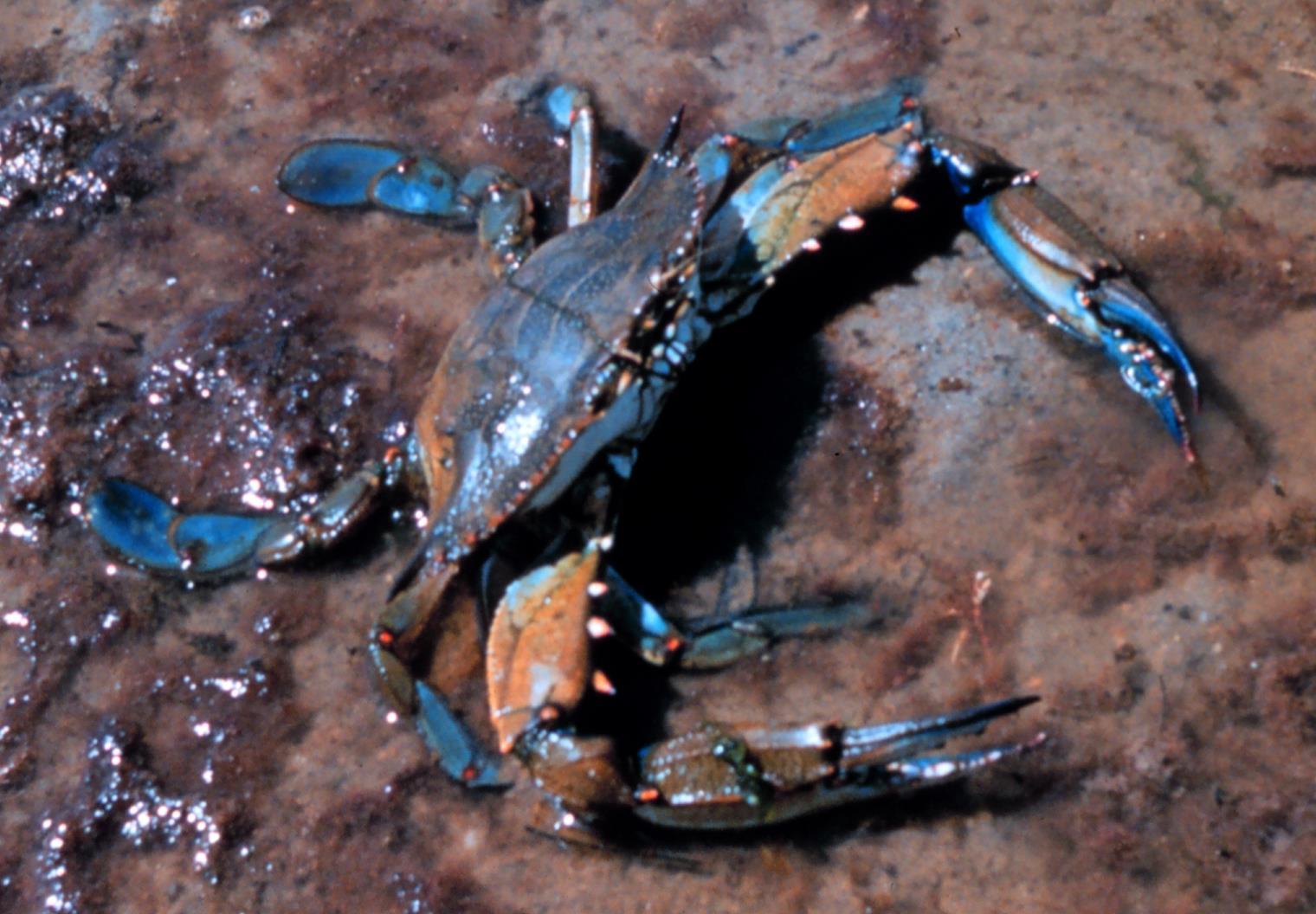
Source: NOAA
Striped Bass
As one of Chesapeake Bay’s top-level piscivores, the striped bass’s interconnected food web indicates that MP exposure has a trickle effect from many sources (Figure A.2-3). Striped bass are at risk of both direct ingestion of MP and indirect exposure to MP through consumption of prey where MP accumulation has occurred (Setälä, Fleming-Lehtinen, and Lehtiniemi 2014). Once these smaller organisms are consumed as prey, larger organisms such as the striped bass are exposed to several different pools of MP in the environment, based on their feeding variability (Murphy et al. 2021). Indirect trophic transfer pathways of MP to striped bass via predation are 3–11 times greater than passive uptake from the water column through the gills, demonstrating the compounding effect of food web concentration through trophic transfers (Hasegawa and Nakaoka 2021).
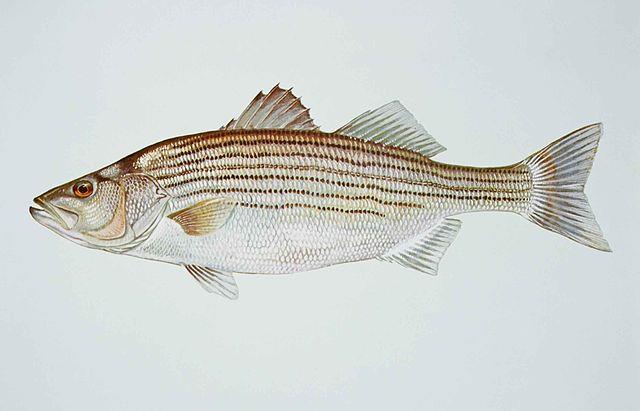
Figure A.2- 3. Striped bass.
Source: Duane Raver, U.S. Fish and Wildlife Service
Striped bass’s increased exposure to MP may contribute to their overall decreased growth and survival. First, MP are known to cause physical blockage of the gut, reducing feeding ability and desire (Murphy et al. 2021), and ultimately leading to potential starvation due to a plastic-filled gut. A study conducted by Lei et al. showed that MP caused intestinal and liver damage in various other fish species, including the cracking of villi, the splitting of the intestinal lining, and disturbance of lipid and energy metabolism (Lei et al. 2018). Along with changes in metabolism, the physical presence of MP can cause other behavioral shifts, such as buoyancy or swimming ability, which can be physically limiting to striped bass and increase their susceptibility to predation (Murphy et al. 2021). Furthermore, MP carry toxic chemicals such as PCBs and PCDEs that can contaminate the fish’s body, having potentially fatal effects on striped bass and their larvae (Du et al. 2020).
Policy Recommendations and Proposed Solutions
The conceptual model for MP risk assessment on hooked mussel, blue crabs, striped bass, and other ecological endpoints demonstrates the still wide-ranging lack of data regarding MP quantities and distribution in the environment, and biological interactions (Tetra Tech 2021). Several actions are needed, locally and federally, to mitigate and hopefully reverse the damaging ecological effects of MP. Recommended actions include:
- Design and implement an MP monitoring program that integrates into the Chesapeake Bay’s existing watershed monitoring infrastructure and framework.
- Develop a comprehensive life cycle analysis of MP pollution from production to deposition.
- Facilitate research to gain greater comprehension of MP pathways in the bay and in its riverine estuaries, such as the Potomac River.
- Ensure adequate infrastructure resources are available to process and analyze MP samples.
- Direct research, management, and policy development to restore ecological habitats and species populations, including ecotoxicological impacts (Murphy et al. 2021).
The management and reduction of plastic pollution, restoration of marine environments, and protection of key species are vital given that global MP production and disposal have been steadily increasing over the past 60 years and about 11% of plastic waste makes its way into waterways and coastal systems (Borrelle et al. 2020, Murphy et al. 2021).
A.3. Impact of Disposable PPE and Single-Use Plastic Items During the COVID-19 Pandemic
Introduction
Between its recognition in 2019 and May 2022, the global SARS-CoV-2 pandemic has caused an estimated 6.2 million global deaths from COVID-19 and has caused a previously unprecedented use of disposable personal protective equipment (PPE), including face masks, face shields, gloves, and gowns, and single-use plastic items for medical use, including disposable wipes, syringes, and sanitizer containers (New York Times 2022), as well as personal use, such as takeout containers and plastic bags. At the start of the pandemic, when uncertainty and fear drove decision-making, individuals flocked to supermarkets, stocking up on single-use utensils, plates, toilet paper, and canned or frozen foods, all containing plastic materials. Concerns about the transmission of SARS-CoV-2 from reusing plastic equipment allowed the emergency relaxation of earlier regulatory and policy efforts to reduce single-use plastics (Prata, Silva, et al. 2020). The use of PPE, in particular face masks, was required by many countries during the height of the pandemic (Li et al. 2022). The waste PPE provides another significant plastic load to the environment both directly by the disposed plastic waste and indirectly by the relaxation of prohibitions against other single-use plastic items (Nghiem, Iqbal, and Zdarta 2021, Patrício Silva, Prata, Walker, et al. 2021). With lockdown measures, social distancing, and public gathering restrictions, citizens have become greatly dependent on online shopping and food takeout and the need for plastic packaging (Figure A.3-1).
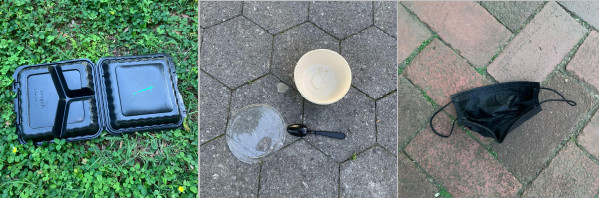
Figure A.3- 1. Examples of single-use plastics.
Source: Caroline Huang
Global Estimates of Single-Use PPE and Plastics Waste
According to Peng et al., the primary waste stream of waste PPE is from hospitals and medical facilities; home disposal of individuals’ PPE was a much smaller waste stream (Peng et al. 2021). PPE includes disposable face masks, gloves, and isolation gowns. Many of these items are made of polypropylene. It is estimated that over 4 million tons of polypropylene waste have been generated since the start of the pandemic (Nghiem, Iqbal, and Zdarta 2021). Prata, Silva, et al. (2020) reported global use estimates of 129 billion face masks and 65 billion gloves monthly. Benson, Bassey, and Palanisami (2021) estimated that, since the start of the pandemic, 3.4 billion single-use face masks or face shields were disposed of daily and plastic waste generated equaled 1.6 million tons/d. Li et al. (2022) estimated that 449.5 billion face masks were used from January 2020 to March 2021.
Data collection from a self-report study taken from February to April 2021 surveyed 202 individuals from 41 countries asking correspondents about a possible change in their consumption of single-use plastics during lockdown (Leal Filho et al. 2021). Fifty-eight percent of respondents indicated their consumption increased, most notably with a 50% increase in food packaging and 35% increase in single-use plastic bags in households (Leal Filho et al. 2021). On a larger scale, prior to the COVID-19 pandemic, plastic pollution totals were already climbing in terrestrial, marine, and atmospheric environments. This growth trend can be applied globally as well, with Peng et al. showing that as of August 2021, 8.4 ± 1.4 million tons of pandemic-associated plastic waste have been generated from 193 countries, with 25.9 ± 3.8 thousand tons released into the global ocean (Peng et al. 2021). Additionally, with safety concerns related to COVID-19 continuing across the globe, plastics demand is expected to increase by another 20%–54% for packaging and 17% for other applications, such as medical uses like PPE (Prata, Silva, et al. 2020). Public concerns are especially evident in food shopping at supermarkets, with customers and providers opting for fresh food packaged in plastic containers and the use of single-use food packaging and plastic bags to avoid contamination (Patrício Silva et al. 2020)
Adverse Effects of Improperly Disposed Plastics and PPE
PPE Litter
Improper disposal of PPE, primarily composed of used face masks, has been reported throughout the world. Littered PPE has been reported all over the world as described by Patrício Silva et al., including beaches in Africa, coastal cities in South America, and cities in Europe (Patrício Silva, Prata, Walker, et al. 2021). River outlets in Jakarta, Indonesia, have been reported to have “unprecedented plastic-made personal protective equipment,” and “abandoned COVID-19 personal protective equipment” has been reported along the Bushehr coastlines of the Persian Gulf (Akhbarizadeh et al. 2021, Cordova et al. 2021). Peng et al. modeled that PPE as litter accumulated along coastlines and was particularly problematic when two conditions were met: proximity to urban communities with large populations and nearness to river mouths and deltas (Peng et al. 2021). Coastal PPE refuse impacts ocean systems through the breakdown of macroplastic waste (particles >5 mm) to MP wastes (particles <5 mm) that easily enter the marine environment. Shen et al. reported that disposable face masks became fragile and easily fragment (Shen, Zeng, et al. 2021). Face mask refuse may contain various dyes and chemical additives, such as plasticizers, stabilizers, antioxidants, antimicrobials, and heavy metals, that can leach into the environment (Li et al. 2022).
Human Health
MP contamination of human blood and MP presence in human lungs have been reported through normal exposure to the environment (Amato-Lourenço et al. 2021, Leslie et al. 2022). Kutralam-Muniasamy, Pérez-Guevara, and Shruti (2022) identified PPE as a complex threat given its ability to generate plastic pollution at the macro-, meso-, micro-, and nano-size levels and the multiple pathways for exposure. Given that disposable face masks are preferentially made from thermoplastics and are worn for long periods of time, concern about the inhalation of micro(nano) plastics shed from the masks themselves has been articulated (Kutralam-Muniasamy, Pérez-Guevara, and Shruti 2022).
The characterization of micro- and nanoplastic particle inhalation risks from face mask use is an active area of study. Han and He noted that face masks standards include requirements for performance and flammability, but no requirements for the allowable amount of respirable debris (micro- and nanoparticles) generated through use are part of the current standards (Han and He 2021). These authors identified the lack of comprehensive information on micro(nano)plastic inhalation risks from face mask use as both a research and a regulatory gap (Han and He 2021). L. Li et al. performed breathing simulation experiments with seven types of commonly used face masks composed of a variety of materials and with the face masks subjected to common cleaning and sterilization treatments for reuse to determine the amount of fiber-like and spherical MP particles inhaled (Li, Zhao, et al. 2021). Because suspended atmospheric MP may be in high concentrations in some environments, Li, Zhao, et al. (2021) concluded that MP inhalation risk was high with or without masking. The MP inhalation risk increased for fiber-like MP as the time of wearing increased (from 2 h to 720 h) for most masks, but the MP inhalation risk for spherical-like particles decreased with time. If masks were treated using common disinfection methods, fiber-like MP inhalation increased. The damaging of mask materials during disinfection treatments was proposed as the reason for the generation of additional respirable particles (Li, Zhao, et al. 2021). The N-95 masks studied provided the lowest inhalation of MP even compared to the no-mask control. There are different methodologies for assessing face mask performance and determining MP shedding; standardization of experimental sampling techniques so inhalation data can be compared has been suggested (Torres-Agullo et al. 2021)
Wildlife and Ecosystem
Improperly disposed of PPE and single-use plastic waste represent a large and additional plastic load to the environment. At the macroscopic level (whole PPE and macroscopic particles >5 mm), terrestrial wildlife has been reported to be affected by ingestion of whole or partial MP litter and by entanglement as plastic pollution continues to fragment and deteriorate into micro- and nano-sized particles. Species reported to have been affected include swans, coots, penguins, shore crabs, bats, hedgehogs, and macaques (Li et al. 2022, Patrício Silva, Prata, Mouneyrac, et al. 2021). Once MP enter the organism’s body, they can cause internal abrasions and blockages and act as a vector of different contaminants, invasive species, and pathogens (Monteiro, Ivar do Sul, and Costa 2018).
Policy Recommendations and Proposed Solutions
- Optimize plastic waste management: A process for gathering and communicating accurate data about the quantity and type of plastic waste and its ability to be reused, recycled, and properly decontaminated should be developed. This process will allow a better determination of the plastic waste type and quantities that are incinerated or landfilled. This information will be crucial for state and federal governments to set valid plastic waste management goals and to regulate the waste management of companies and individuals (Hartmann et al. 2017).
- Implement sustainable safety measures: The use of reusable grocery and shopping bags should be encouraged with simultaneous public education on proper hand hygiene and decontamination methods for reusable bags (for example, soaking the bags in a decontamination bath of liquid soap and water >40°C). Practice of safety measures also applies to home deliveries, and service workers should be trained by their employers to wear protective equipment and frequently sanitize their hands. Additionally, corporations should implement an in-person drive-through window to facilitate online shopping, ordering, and pickup (Patrício Silva, Prata, Walker, et al. 2021).
- Collaborate between academia and government to increase public awareness: Better integration of academic and government activities is suggested to promote sustainable production, use, and disposal of PPEs and plastics by financially backing scientific research in these areas. In addition, academic-government collaborations will be important in the development of relevant communication channels to increase the public’s awareness of plastic pollution. This integration would require more research and monitoring programs, including citizen science programs, to survey and collect data on environmental PPE load (Patrício Silva, Prata, Mouneyrac, et al. 2021, Tesfaldet and Ndeh 2022). The creation of synergisms between academia and government by creating communication forums and other public participatory channels will raise awareness and educate on the proper collection and disposal of used plastics and PPE, with the goal of long-term behavioral changes (Patrício Silva, Prata, Walker, et al. 2021, Prata, Silva, et al. 2020, Shams, Alam, and Mahbub 2021).
Given that the current UN estimate for global human population in 2050 is 9.8 billion persons and global pandemics are part of humanity’s future, the management and proper disposal of plastic PPE and single-use plastics is necessary. Relaxation of single-use plastic restrictions due to the 2019 pandemic response should be ended and plastic use minimization resumed.
A.4. Nurdles along the Gulf Coast
Nurdles are small preproduction plastic pellets that are used as raw materials in plastic product manufacturing (Figure A.4-1). Measuring less than 5 millimeters in size, nurdles are considered primary MP and are the second largest source of ocean MP. Nurdles can be made of PE, PP, PS, PVC, or other polymers and include various chemical additives (Tunnell et al. 2020). These pellets can be released during production or in transit and have been found along beaches and in waterways across the country, including in the Gulf Coast region. The Gulf Coast is home to offshore petroleum production and on-shore petrochemical plants. Major rivers such as the Mississippi River are connected to the Gulf Coast, and these waterways are used for shipping and transportation of various cargo, including nurdles. It is estimated that 200,000 metric tons of nurdles have been released into oceans (Pew Charitable Trust 2020). Because nurdles are not classified as pollutants or hazardous materials, there is little regulatory push to respond to these spills or discharges (Dhanesha 2022). Although the Coast Guard is typically responsible for oil or other toxic substances released in waterways, the Coast Guard has stated they do not have jurisdiction over nurdles and are not responsible for cleanups (Baurick 2020).
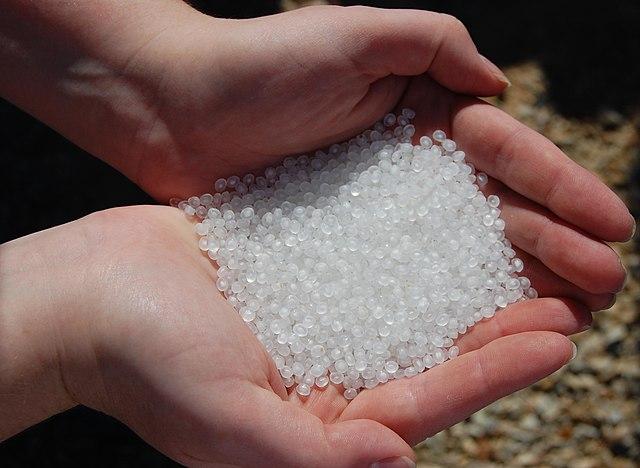
Source: gentlemanrook / CC-BY-2.0
Nurdle Spills
A notable nurdle spill occurred in New Orleans in 2020, in which a cargo ship spilled millions of nurdles into the Mississippi River (Baurick 2020). The nurdles were released when the cargo ship broke free from its moorings during a storm, and a large container of nurdles was released into the river. The submerged container was retrieved from the river 2 days after the storm, and sacks of nurdles fell out of the damaged container. The area of release is up to 200 feet deep, and it was determined that the pellets were irretrievable. This spill illustrates one of the many ways that nurdles are released into waterways.
Formosa Plastics Lawsuit
In June 2019, a Texas judge found Formosa Plastic Corporation, a petrochemical company, in violation of the Clean Water Act for discharging millions of nurdles into Cox Creek and Lavaca Bay, Texas (United States District Court for the Southern District of Texas 2019). The evidence for the Formosa case was primarily built on data collected by a citizen science group, the San Antonio Bay Estuarine Waterkeeper. Led by Diane Wilson, a retired shrimp boat captain and fisherwoman, volunteers with the group combed the Gulf Coast to document nurdles in the environment via photographs and videos and collected thousands of nurdle samples (Berti Suman and Schade 2021, Gardiner 2022). This effort started in January 2016 and continued for over 3 years. The lawsuit against Formosa was filed in 2017, and the ruling was delivered in 2019. Formosa agreed to pay $50 million over 5 years in mitigation payments that would fund various projects aimed at revitalizing the beaches and waterways that were affected by the nurdle discharge. As part of the settlement agreement, Formosa also agreed to zero-discharge plastic production, and if found in violation again, the company would be required to pay additional penalties for each discharge. Since the settlement, Formosa has already accumulated millions of dollars in new fines.
The Formosa Plastics settlement is the largest settlement of a Clean Water Act lawsuit filed by private citizens, and it has set a precedent for other similar suits by environmental organizations in response to the lack of nurdle pollution regulations. A similar case in Charleston, South Carolina, between Charleston Waterkeeper and Frontier Logistics, LP, a plastics distribution company, resulted in a settlement of $1 million paid by Frontier (Post and Courier Editorial Staff 2022)
Public Awareness and Citizen Science
A citizen science group called Nurdle Patrol was started in 2018 by the Mission-Aransas National Estuarine Research Reserve at the University of Texas Marine Science Institute in Port Aransas, Texas, with the goal of mapping nurdle locations, removing released nurdles from the environment, and educating the public about nurdles (Nurdle Patrol 2022). The group relies on volunteers and citizens to complete nurdle surveys, which consist of counting nurdles on beaches in a ten-minute time frame. The quantity and location data are then uploaded to the Nurdle Patrol database and outputted as an interactive map. Nurdle Patrol partners with nurdle tracking groups across the United States and across 14 countries; as of September 2021, over 10,000 nurdle surveys had been completed. Nurdle Patrol documented close to 1.8 million nurdles between November 2018 and May 2022. Nurdle Patrol has expanded from nurdle counts to also participating in education outreach and research initiatives, such as fingerprinting the chemical makeup of nurdles. Figure A.4-2 shows an example of a nurdle count as well as the interactive mapper showing the number of nurdles collected.
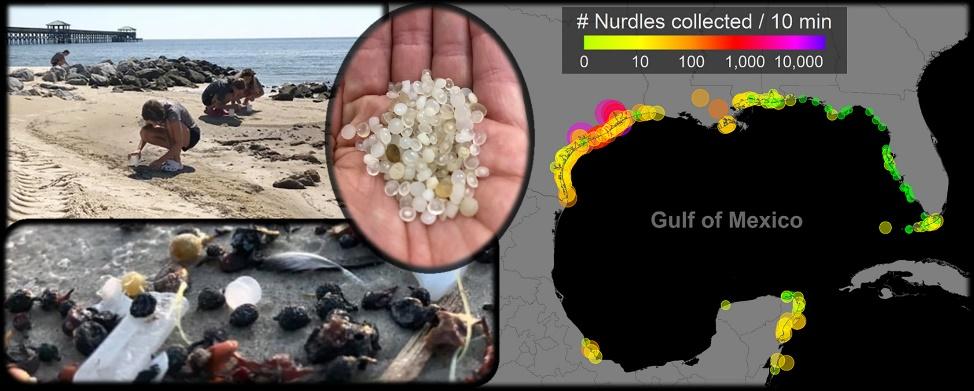
Figure A.4-2. Nurdle Patrol and Gulf Coast nurdle map.
Source: Tunnell et al. (2020)
Conclusion
The volunteer efforts by groups such as the San Antonio Bay Estuarine Waterkeeper and Nurdle Patrol in the Gulf Coast are examples of how citizen science can play a significant role in understanding and evaluating emerging contaminants, as well as drive litigation, which can ultimately impact policy. The data generated by citizen science groups can be used to inform decision-maker responses and support ongoing research efforts on understanding and mitigating nurdle pollution.
A.5. Effects of 6PPD-quinone on Coho and Chum Salmon
This case study will detail the impact tire wear particles (TWP) are having on coho salmon (Oncorhynchus kisutch) in the Pacific Northwest of the United States. TWP are generated from the friction between tires and road surfaces and can deposit in near-roadway surface water and soil. These particles can also deposit in stormwater control systems near road surfaces, providing a potential transport pathway for the particles to reach surface waters. These TWP contain leachable chemical additives from the tread, including an antiozonant called N-(1,3-dimethylbutyl)-N′-phenyl-p-phenylenediamine (6PPD). The ozonation byproduct of 6PPD, 6PPD-quinone, has been linked to coho salmon mortality. At the current rate, approximately 3.1 billion tires are manufactured annually. It is challenging to identify and analyze the composition of TWP given their complex and variable properties (for example, density, particle size, polymer content). However, a study by the San Francisco Estuary Institute (SFEI) was able to identify one particle matching tire rubber using Raman spectroscopy (Sutton et al. 2019, Werbowski et al. 2021). Approximately 0.81 kg of tire particles are emitted each year per capita worldwide, posing a threat to organisms that may encounter these tire particles. Therefore, aquatic organisms, including species of salmon, face potential adverse health effects from TWP, including potentially leachable chemicals (Figure A.5-1).
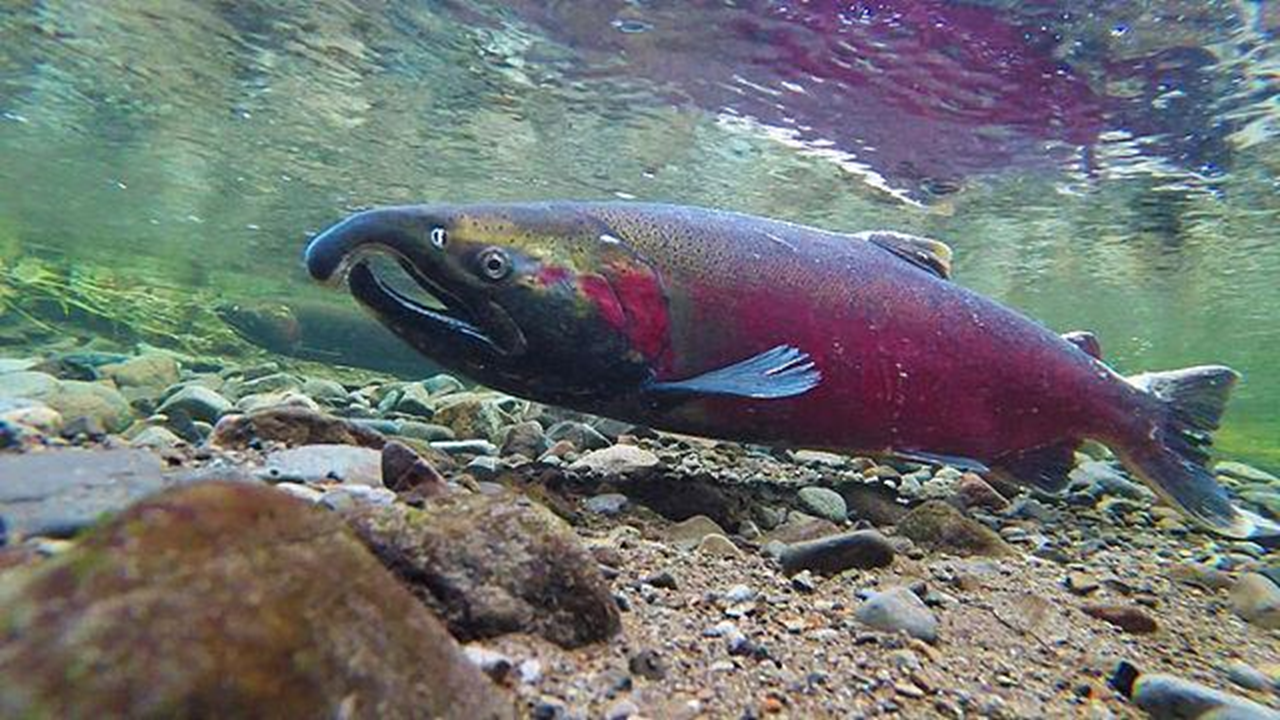
Figure A.5- 1. Coho salmon.
Source: Bureau of Land Management
Objectives
The objective of this study was to evaluate TWP impact on acute mortality in coho and chum salmon. This case study will also detail mitigation efforts that can be made to decrease the transport of tire particles to surface waters, as described by the SFEI.
Results
A study published by Environmental Science Technology Letters in 2022 detailed the account of tire rubber-derived chemicals causing mortality issues in coho salmon (Oncorhynchus kisutch). In this study, it is estimated that 40%–90% of coho salmon attempting to respawn will die in an urbanized watershed area (Tian et al. 2021). The study analyzed water samples from three different Seattle watersheds before a storm, during a storm, and after the storm. Pre- and post-storm samples did not present any evidence of 6PPD. However, samples collected during the storm maintained a concentration around the LC50 limit (Tian et al. 2021). To clarify, the LC50 limit is the concentration of a chemical that has the potential to kill 50% of a sample population. This is evidence of a potentially dangerous scenario for marine organisms that come into contact with 6PPD during a storm event, as it can cause runoff that can reach nearby water bodies.
A separate study published in Environmental Science and Technology (McIntyre et al. 2021) provided further evidence of acute mortality rates in coho salmon exposed to TWP leachate that contained 6PPD-quinone. Adult coho salmon exposed to 100 mg TWP/L showed mortality rates between 25% and 50% for an exposure period of 24 hours. There was a 100% mortality rate for adult coho salmon exposed to 320 mg TWP/L during 24 hours of exposure. However, the same results were not found among chum salmon; there was a 0% mortality rate after exposure to 320 mg TWP/L for 24 hours (McIntyre et al. 2021)
Solutions
There are several ways to mitigate the transport of TWP to the environment and ultimately the effects TWP have on environmental health (Moran et al. 2021). First, innovations in reducing tire wear rate and tire particle emissions will significantly decrease the amount of TWP entering waterways. Second, alternative chemicals may be used in tire formulations, which will require extensive research initiatives such as alternative assessments (for example, DTSC Safer Consumer Products). Third, improved collection and treatment technologies could be implemented to manage TWP transport throughout the environment (for example, porous road surfaces, stormwater or runoff collection). Further research must be conducted to study the biological differences between the coho salmon and the chum salmon species to determine why coho salmon is more susceptible to mortality caused by exposure to 6PPD-quinone than chum, and expanded research is also needed on TWP toxicity on other aquatic organisms impacted by surface water.
A-6. Washing Machine Filters Reduce Microfiber Emissions to Aquatic Ecosystems
This policy brief presents information on using washing machine filters to remove microfibers from washing machine emissions. AppendixA6.pdf